insights
Environmental Toxicants: A Risk Factor for Neurodegenerative Diseases
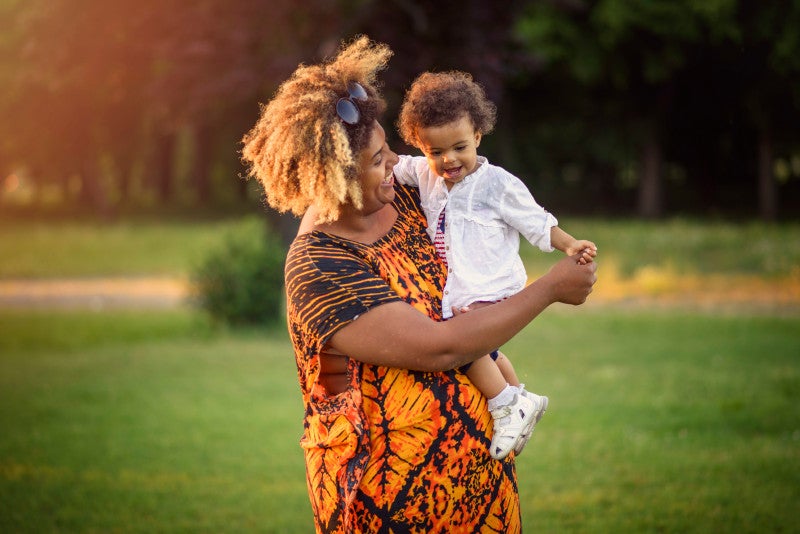
Learn proven functional medicine strategies for treating toxic exposures at the upcoming Environmental Health Advanced Practice Module. SEE FULL PROGRAM DETAILS
Read Time: 8 Minutes
As world populations grow older and fertility rates decline, leading to a simultaneously shrinking and aging society, the incidence of neurodegenerative diseases is projected to become even more pervasive than it is today.1 Globally, people above 65 years old are the fastest-growing segment of the population; in 2018, for the first time in human history, they outnumbered children younger than five years old.2 In the US alone, the incidence of Alzheimer’s disease could more than double by 2050 to 13.8 million, from 5.8 million today.3 China has the largest number of Parkinson’s disease patients in the world, which is estimated to grow to five million in 2030.1 In light of these statistics, research into the connection between environmental toxins and neurodegenerative disease is more important than ever.
While genetics has a known causative role, researchers agree that environmental risk factors can also play a key role in accelerating disease onset and progression.4 Neurodegenerative diseases are complex and multifactorial, encompassing a variety of conditions, each with different pathological patterns, clinical presentations, and root causes.5,6 With regard to the most common neurodegenerative diseases, Alzheimer’s disease (AD) and Parkinson’s disease (PD), the most rigorously studied environmental exposures have been on heavy metals and pesticides.7
Alzheimer’s Disease, Dementia, & Heavy Metals
Across the globe, over 50 million people suffer from dementia, and this number is expected to reach 152 million by the year 2050.8 AD development, which is clinically manifested by progressive impairment in cognition, learning ability, memory function, and executive reasoning,9 is partially attributable to environmental factors.8 Heavy metals like lead, cadmium, and manganese are widely used and may contribute to AD pathologies by increasing neuronal oxidative stress, inflammation, and apoptosis.5,8
Lead
Despite US legislative efforts to minimize lead exposure, this heavy metal is still used in industrial applications, including automobile lead-acid storage batteries.8 Lead exposure sources tend to vary by geographic location; however, in general, globally high lead levels are associated with electronic waste recycling, lead mining, and smelting, with the primary routes of exposure being inhalation or ingestion. Particularly concerning is lead exposure in children, which increases the body burden, as lead dust is often ingested due to a child’s frequent hand-to-mouth behavior.8 Longitudinal studies suggest that early-life or mid-life lead exposure may be associated with faster rates of cognitive decline.4,8 Animals exposed to lead (either prenatally or postnatally) develop memory impairment and cognitive decline later in life.4
Lead is a known neurotoxicant that rapidly crosses the blood-brain barrier,4 leading to neuroinflammation, oxidative stress, endoplasmic reticulum stress, and apoptosis.8 It has been associated with neurodegeneration in cross-sectional human epidemiology studies,8,10,11 and numerous studies have reported that either developmental or acute lead exposure contributes to the hallmark signatures of AD, including A? accumulation, tau pathology, and inflammation.4 Lead poisoning may also be accompanied by inflammatory events that lead to neuronal death.4 Recent studies suggest that lead exposure results in the activation of microglia and the overproduction of proinflammatory proteins such as inducible nitric oxide synthase (iNOS), interleukin-1 beta (IL-1?), and tumor necrosis factor alpha (TNF-?), and these factors are known to contribute to neurotoxicity in AD.1,4
Cadmium
Cadmium has recently emerged as a neurotoxicant, although evidence in humans remains limited.8 Diet is the primary exposure source, along with cigarette smoking. Like lead, cadmium is known to cross the blood-brain barrier; once in the body, this metal may induce oxidative stress, neuroinflammation, and apoptosis in neuronal cells.4,8 A 2023 systematic review of observational studies found that among older adults, cognitive ability scores decreased as measured levels of blood, urine, and dietary cadmium increased.1
Recent epidemiological studies reported that blood cadmium levels were significantly associated with AD-related mortality among older adults.4,13,14 A meta-analysis including eight studies covering 405 AD patients and 424 control subjects found that circulating concentrations (either whole blood, serum, or plasma) of cadmium were significantly higher in AD versus controls.8,15 The authors note that the findings from postmortem brain tissues are subject to confounding by AD risk factors, especially age.8,15 A cross-sectional study with 2,068 older adults from the US National Health and Nutrition Examination Survey 2011-2014 showed a significant association between cadmium exposure measured in whole blood and lower cognitive function.8,16
Manganese
Despite the importance of manganese in human health (it is a cofactor for normal cell function enzymes), excessive manganese is neurotoxic, as high levels may cause accumulation in the brain.8 Beyond occupational exposure, diet is the primary source of manganese in the general population, and toxicity can also result from elevated levels in drinking water or air; it is widely used in industrial processes and commercial products.17 Research suggests that ingested manganese has an absorption rate of 3-5% through the gastrointestinal tract and is subject to tight homeostatic control in vivo.17 Several factors may influence the oral uptake of manganese, including iron status, diet, bioavailability, and existing body burden.17 Underlying mechanisms include induction of oxidative stress, mitochondrial dysfunction, autophagy dysregulation, accumulation of intracellular toxic metabolites, and apoptosis.4,8 Recent bioinformatics analysis suggests that manganese exposure may induce the differential expression of genes related to a cytokine-cytokine receptor interaction, apoptosis, oxidative phosphorylation, toll-like receptor signaling pathway, and insulin signaling pathway.4,18
Epidemiologic studies using hair, blood, or both hair and blood biomarkers report associations between adult manganese levels and impaired cognitive function.8,17 A 2022 systematic review of observational studies conducted in low to upper-middle income countries reported negative associations between manganese levels and neurodevelopment domains in children, including attention, memory, learning, and executive function.19 Those with occupational exposure have reported deficits in processing speed, attention, memory, reaction time, cognitive control, and other cognitive functions.20 Research suggests that accumulation of manganese in the liver and the brain, particularly in the basal ganglia, likely causes neurotoxicity and hepatic damage.17
Parkinson’s Disease & Pesticides
Parkinson’s disease (PD) is a common neurodegenerative disease, second worldwide only to Alzheimer’s disease, with approximately 60,000 new cases diagnosed in the US each year.21 PD is characterized by motor symptoms, including bradykinesia plus rigidity and resting tremor, as well as postural instability at a more advanced stage.21 It may also be coupled with non-motor features such as dementia, depression, and autonomic dysfunction.9 A 2019 systematic review and meta-analysis suggests that exposure to pesticides increases the risk of developing a neurodegenerative disease, including PD, by 50%.22 Rural living and occupational exposure to pollutants and pesticides are additional exacerbating factors.23
Decades of epidemiological and observational studies suggest a link between insecticide exposure through ingestion or skin contact and incidence of PD.24-26 A recent meta-analysis indeed shows that epidemiologic data generally support this correlation.27 Exposure to paraquat or maneb/mancozeb in particular was associated with about a two-fold increase in risk. In high-quality case-control studies, PD risk was increased by exposure to any type of pesticides, herbicides, and solvents; also, the risk associated with rural living was found to be significant.27
Another fascinating 2020 occupational study was based on access to 23,224 patient hospital records with the primary diagnoses of PD and allowed the mapping of cases against known crop distributions and pesticides in Louisiana.21 Major PD-affected areas were associated with commercial forests, woodlands, and pastures and associated with certain pesticides, including 2,4-D, chlorpyrifos, and paraquat. The authors report that the extensive rural areas overlay the areas of significant potential aquifer recharge within Louisiana, which would have ensured human exposure whenever the pesticides were deployed.21 (Note: aquifer recharge is water that moves from the land surface or unsaturated zone into the saturated zone. Where contamination of an aquifer is a concern, estimating the recharge rate is a first step toward predicting solute transport to the aquifer).
The group of pesticides known as organochlorines are most frequently associated with the risk of PD, as they have consistently been shown to be neurotoxic and to promote oxidative stress.23 One of the first studies to measure actual pesticide levels in a case control study setting, Dardiotis et al in 2020, was a study of a Greek PD cohort living in a rural area where the pesticide exposure was expected to be high. All eight organochlorine pesticides studied were present in at least one sample. The most frequently detected pesticides were p,p’-DDE (n=214, 100% of both groups) and hexachlorobenzene, or HCB (n=189, cases 46.5%, controls 53.5%). Other studies have also linked DDT and systematic exposure to pesticides to an elevated risk of developing PD; its extremely long half-life facilitates bioaccumulation, biomagnification, and its transport through the blood-brain barrier and into the brain parenchyma. DDT has also been known to cause mitochondrial dysfunction, a process involved in PD pathogenesis.23
Mechanisms of Action: New Research Into Neuronal Cells
A considerable amount of evidence demonstrates that AD and PD share common clinical and neuropathologic features, and that overlap between the two conditions is extensive.28 Many mechanisms involved in AD and PD may be the same, such as genes, ?-synuclein protein, tau protein, oxidative stress, and mitochondrial dysfunction.28 And while the exposure to environmental toxicants like heavy metals in AD and pesticides in PD have been implicated, their impact on astrocytes represents an expanding area of research.28,29
Astrocytes, the most numerous cell type, are critical for proper brain function in part due to their robust antioxidant and unique metabolic capabilities.29 They are positioned both at and in the blood-brain barrier, where they are the primary responders to xenobiotic penetrance of the central nervous system, and they are critical for the maintenance of lifelong brain health.29
Some researchers believe that the response of astrocytes to environmental toxicants is crucial to understanding the impact on the brain.29 Specifically, astrocytic metabolic dysfunction as a result of both acute and chronic exposure to toxicants may contribute to the breakdown of homeostasis and attendant neural degeneration.29 For example, manganese exposure results in cell swelling in cultured astrocytes, a morphological change also found in AD.29 Intracellularly, excess manganese interferes with a variety of cellular processes, and impairment of astrocytic metabolic processes by manganese toxicity results in both direct neuronal dysfunction as well as secondary dysfunction derived from compounding effects. Furthermore, disturbances to astrocytic mitochondrial homeostasis and their ability to produce ATP can negatively impact neural function.30
Evidence that astrocytic metabolism is important for the response to and neutralization of environmental toxicants within the central nervous system is growing.29,30 While most of the research to date focuses on neuronal damage in cases of age-related neurodegeneration like AD and PD, there is a body of evidence that supports a central mechanistic role for astrocyte mitochondria in the expression of neural injury.30 As research evolves, astrocytes might become a new target for neurodegenerative disease therapeutics.
Interventions
Understanding toxicity and taking practical steps to improve biotransformation and the elimination of toxicants are essential and critical pieces in the functional medicine approach to health and well-being. Functional medicine educates clinicians about the biochemistry and genetics of biotransformation pathways, the connection between organ system dysfunction and potential toxic exposures, the laboratory evaluations necessary in working up a toxin-exposed patient, and various personalized treatment approaches. Treatments for patients concerned about toxic exposures may include support of mitochondrial health and concurrent consideration of multiple lifestyle factors, including nutrition and exercise.
Probiotic Therapies
Some probiotics possess antimicrobial abilities, and studies suggest that they may also reduce pathogenic toxins and produce antimicrobial metabolites (short-chain fatty acids, bacteriocins, reuterin, linoleic acid, and secondary bile acids) for enhancing intestinal or systemic immunity.31 In this respect, they may be used to alleviate heavy metal toxicity, as antioxidative defense and a healthy immune system are essential to maintaining human health.31 Recent research suggests that the use of food-grade and probiotic lactobacilli to reduce the absorption of heavy metals may be a safe, simple, and affordable solution for reducing exposure.32,33
For example, Lactobacillus rhamnosus GR-1 (LGR-1) was shown to reduce heavy metal bioaccumulation in a Tanzanian cohort of women and children.32 The authors hypothesized that LGR-1 could sequester the heavy metals lead and cadmium, thereby reducing their absorption across intestinal epithelium. LGR-1 and other lactobacilli significantly reduced the amount of Pb and Cd in solution at all concentrations tested (0.5 mg/L–50 mg/L) and exhibited sustained binding profiles over a 48-hour period. In this in vitro study, LGR-1 was able to significantly reduce apical-to-basolateral translocation of Pb and Cd in a Caco-2 model of the intestinal epithelium.32
Nutrition
Specific nutrients and dietary patterns have been investigated for their neuroprotective properties. From reducing inflammatory markers and severity of symptoms to improving outcomes and quality of life, nutrition is an essential part of a patient’s personalized clinical intervention for neurodegenerative diseases. A functional medicine strategy for prevention and treatment of neurodegeneration may include a therapeutic food plan such as IFM’s Detox Food Plan, which benefits patients by helping them eat more of the foods that support pathways in the liver for healthy elimination. In functional medicine, practitioners often utilize the phytonutrient-dense Detox Food Plan to support intestinal and liver function during the metabolic detoxification process.
Research suggests that a diet rich in antioxidants like fruits, nuts, vegetables, and spices reduced caloric intake, and a low consumption of alcohol may lower age-related cognitive decline and lessen the risk of developing neurodegenerative diseases.34 More recent research supports the role of B vitamins, vitamin D, folate, polyphenols, and n-3 polyunsaturated fatty acids in neuroprotection and in reducing the risk of cognitive decline.34,35 Additionally, cooking methods should be considered as some procedures can generate potential toxic compounds such as advanced glycation end products that contribute to the aging process and neurodegenerative diseases.34
Certainly, avoiding toxic exposures is key to optimal wellness, but in today’s society, it has become increasingly challenging to do so. Given the presence of a vast array of chemicals that humans may be exposed to in their lifetime, how do we ensure human health? This is the focus of IFM’s Environmental Health Advanced Practice Module. An individual’s ability to detoxify or biotransform and excrete toxic substances is of critical importance to overall health. Learn how to assess both exposures and total toxic load to appropriately assess and address each individual’s toxicological situation.
Related Articles
Detoxification: Supporting Liver Function With Nutrition
Toxic Environmental Exposures and Energy Production
The Connection Between POPs and Metabolic Syndrome
References
- Liu C, Liu Z, Zhang Z, et al. A scientometric analysis and visualization of research on Parkinson’s disease associated with pesticide exposure. Front Public Health. 2020;8:91. doi:3389/fpubh.2020.00091
- United Nations Department of Economic and Social Affairs. World Population Prospects 2019: Highlights. Published June 2019. Accessed July 10, 2023. https://population.un.org/wpp/Publications/Files/wpp2019_10KeyFindings.pdf
- Mather M, Scommegna P, Kilduff L. Fact sheet: aging in the United States. Population Reference Bureau. Published July 15, 2019. Accessed July 10, 2023. https://www.prb.org/resources/fact-sheet-aging-in-the-united-states/
- Huat TJ, Camats-Perna J, Newcombe EA, Valmas N, Kitazawa M, Medeiros R. Metal toxicity links to Alzheimer’s disease and neuroinflammation. J Mol Biol. 2019;431(9):1843-1868. doi:1016/j.jmb.2019.01.018
- Cicero CE, Mostile G, Vasta R, et al. Metals and neurodegenerative diseases. A systematic review. Environ Res. 2017;159:82-94. doi:1016/j.envres.2017.07.048
- Armstrong RA. What causes neurodegenerative disease? Folia Neuropathol. 2020;58(2):93-112. doi:5114/fn.2020.96707
- Gunnarsson LG, Bodin L. Occupational exposures and neurodegenerative diseases—a systematic literature review and meta-analyses. Int J Environ Res Public Health. 2019;16(3):337. doi:3390/ijerph16030337
- Bakulski KM, Seo YA, Hickman RC, et al. Heavy metals exposure and Alzheimer’s disease and related dementias. J Alzheimers Dis. 2020;76(4):1215-1242. doi:3233/jad-200282
- Cui L, Hou NN, Wu HM, et al. Prevalence of Alzheimer’s disease and Parkinson’s disease in China: an updated systematical analysis. Front Aging Neurosci. 2020;12:603854. doi:3389/fnagi.2020.603854
- Zhao Y, Ray A, Portengen L, Vermeulen R, Peters S. Metal exposure and risk of Parkinson disease: a systematic review and meta-analysis. Am J Epidemiol. 2023;192(7):1207-1223. doi:1093/aje/kwad082
- Farace C, Fiorito G, Pisano A, et al. Human tissue lead (Pb) levels and amyotrophic lateral sclerosis: a systematic review and meta-analysis of case-control studies. Neurol Sci. 2022;43(10):5851-5859. doi:1007/s10072-022-06237-y
- Yang X, Xi L, Guo Z, Liu L, Ping Z. The relationship between cadmium and cognition in the elderly: a systematic review. Ann Hum Biol. 2023;50(1):15-25. doi:1080/03014460.2023.2168755
- Min JY, Min KB. Blood cadmium levels and Alzheimer’s disease mortality risk in older US adults. Environ Health. 2016;15(1):69. doi:1186/s12940-016-0155-7
- Peng Q, Bakulski KM, Nan B, Park SK. Cadmium and Alzheimer’s disease mortality in US adults: updated evidence with a urinary biomarker and extended follow-up time. Environ Res. 2017;157:44-51. doi:1016/j.envres.2017.05.011
- Xu L, Zhang W, Liu X, Zhang C, Wang P, Zhao X. Circulatory levels of toxic metals (aluminum, cadmium, mercury, lead) in patients with Alzheimer’s disease: a quantitative meta-analysis and systematic review. J Alzheimers Dis.2018;62(1):361-372. doi:3233/jad-170811
- Li H, Wang Z, Fu Z, et al. Associations between blood cadmium levels and cognitive function in a cross-sectional study of US adults aged 60 years or older. BMJ Open. 2018;8(4):e020533. doi:1136/bmjopen-2017-020533
- Balachandran RC, Mukhopadhyay S, McBride D, et al. Brain manganese and the balance between essential roles and neurotoxicity. J Biol Chem. 2020;295(19):6312-6329. doi:1074/jbc.rev119.009453
- Ling J, Yang S, Huang Y, Wei D, Cheng W. Identifying key genes, pathways and screening therapeutic agents for manganese-induced Alzheimer disease using bioinformatics analysis. Medicine (Baltimore). 2018;97(22):e10775. doi:1097/md.0000000000010775
- Heng YY, Asad I, Coleman B, et al. Heavy metals and neurodevelopment of children in low and middle-income countries: a systematic review. PLoS One. 2022;17(3):e0265536. doi:1371/journal.pone.0265536
- Vlasak T, Dujlovic T, Barth A. Manganese exposure and cognitive performance: a meta-analytical approach. Environ Pollut. 2023;332:121884. doi:1016/j.envpol.2023.121884
- Hugh-Jones ME, Peele RH, Wilson VL. Parkinson’s disease in Louisiana, 1999-2012: based on hospital primary discharge diagnoses, incidence, and risk in relation to local agricultural crops, pesticides, and aquifer recharge. Int J Environ Res Public Health. 2020;17(5):1584. doi:3390/ijerph17051584
- Gunnarsson LG, Bodin L. Occupational exposures and neurodegenerative diseases—a systematic literature review and meta-analyses. Int J Environ Res Public Health. 2019;16(3):337. doi:3390/ijerph16030337
- Dardiotis E, Aloizou AM, Sakalakis E, et al. Organochlorine pesticide levels in Greek patients with Parkinson’s disease. Toxicol Rep. 2020;7:596-601. doi:1016/j.toxrep.2020.03.011
- Shrestha S, Parks CG, Umbach DM, et al. Pesticide use and incident Parkinson’s disease in a cohort of farmers and their spouses. Environ Res. 2020;191:110186. doi:1016/j.envres.2020.110186
- Priyadarshi A, Khuder SA, Schaub EA, Shrivastava S. A meta-analysis of Parkinson’s disease and exposure to pesticides. 2000;21(4):435-440.
- Yan D, Zhang Y, Liu L, Shi N, Yan H. Pesticide exposure and risk of Parkinson’s disease: dose-response meta-analysis of observational studies. Regul Toxicol Pharmacol. 2018;96:57-63. doi:1016/j.yrtph.2018.05.005
- Pezzoli G, Cereda E. Exposure to pesticides or solvents and risk of Parkinson disease. 2013;80(22):2035-2041. doi:10.1212/wnl.0b013e318294b3c8
- Xie A, Gao J, Xu L, Meng D. Shared mechanisms of neurodegeneration in Alzheimer’s disease and Parkinson’s disease. Biomed Res Int. 2014;2014:648740. doi:1155/2014/648740
- McCann MS, Maguire-Zeiss KA. Environmental toxicants in the brain: a review of astrocytic metabolic dysfunction. Environ Toxicol Pharmacol. 2021;84:103608. doi:1016/j.etap.2021.103608
- Kubik LL, Philbert MA. The role of astrocyte mitochondria in differential regional susceptibility to environmental neurotoxicants: tools for understanding neurodegeneration. Toxicol Sci. 2015;144(1):7-16. doi:1093/toxsci/kfu254
- Tang C, Lu Z. Health promoting activities of probiotics. J Food Biochem. 2019;43(8):e12944. doi:1111/jfbc.12944
- Daisley BA, Monachese M, Trinder M, et al. Immobilization of cadmium and lead by Lactobacillus rhamnosus GR-1 mitigates apical-to-basolateral heavy metal translocation in a Caco-2 model of the intestinal epithelium. Gut Microbes. 2019;10(3):321-333. doi:1080/19490976.2018.1526581
- Abdel-Megeed RM. Probiotics: a promising generation of heavy metal detoxification. Biol Trace Elem Res. 2021;199(6):2406-2413. doi:1007/s12011-020-02350-1
- Agnihotri A, Aruoma OI. Alzheimer’s disease and Parkinson’s disease: a nutritional toxicology perspective of the impact of oxidative stress, mitochondrial dysfunction, nutrigenomics and environmental chemicals. J Am Coll Nutr. 2020;39(1):16-27. doi:1080/07315724.2019.1683379
- Moore K, Hughes CF, Ward M, Hoey L, McNulty H. Diet, nutrition and the ageing brain: current evidence and new directions. Proc Nutr Soc. 2018;77(2):152-163. doi:1017/s0029665117004177